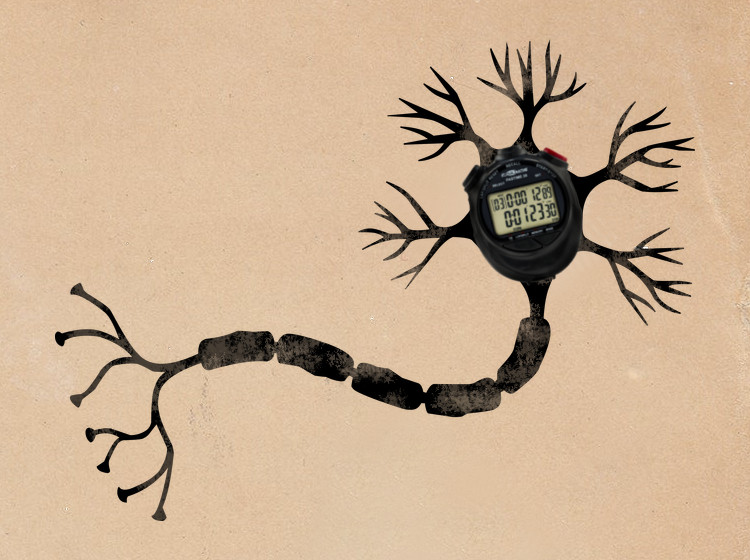
How does the brain keep track of time? This question has been intriguing neuroscientists for decades. Circadian clocks, which oscillate every 24 hours, are known to be implemented at the level of molecules and genes. But it is widely believed that keeping track of time for shorter durations (e.g. seconds and minutes) arise from electrical/synaptic activity patterns, not from molecular activity. The idea is that cells can be connected in ways that result in oscillations or sequential activity (e.g. one neuron fires at the 1s mark, the next fires at the 2s mark, etc.). As with most of our theories of short-term memory, if all the cells in a network go silent for a moment the timer falls apart. The spiking activity is what keeps the clock going. This theory has had its opponents, but I think it is fair to say that it has been a commonly held view in neuroscience.
A recent study, however, has made a serious crack in this paradigm. In a series of two papers from the Crickmore lab at Harvard University (one published last year and another last month), Thornquist and colleagues show that a single neuron can keep track of time in a completely silent manner. The time interval they studied was a 7 minute period in mating fruit flies. I believe this is a landmark study that every neuroscientist should know about. So here is my attempt at explaining it in simple terms.
Fruit flies mate for a very stereotyped 20 minute duration and the male ejaculates at the 7 minute marker. Before ejaculation, the male fly is so motivated to continue that it will even risk death to continue mating. After ejaculation, it is easier to get a male fly to let go of the female using heat or noxious stimuli. So the 7 minute timer not only initiates a specific behavior (i.e., ejaculation) but also controls a behavioral state (i.e., the male’s motivation to continue mating).
Now, it has been known for quite some time that there are exactly four neurons (called the “Corazonin” neurons) located in what would be the equivalent of the spinal cord for fruit flies (in the Drosophila ventral nerve cord or ventral nervous system). These neurons are silent for the most part, but suddenly erupt about 7 minutes after the onset of copulation. At least two of them have to fire at the same time to drive ejaculation and switch the fly’s motivational state. In practice, though, all four fire together at the same time.
The first thought that comes to a neuroscientist’s mind is that “these four neurons are downstream of the central nervous system and they’re silent for the most part. So they’re unlikely to be involved in any complicated computation. They are probably just gatekeepers of the ejaculation event. Some upstream neurons must keep track of time and send excitatory inputs to the Corazonin neurons for the fly to ejaculate“. Right? Wrong!
It turns out that each of these four neurons have their own cell-intrinsic timer! Once a cell’s 7 minute timer is up, it fires. The four neurons are recurrently connected so a cell will also fire if it sees that the other neurons fire.
Imagine four agents sitting around a large table, each with their own small 7-minute hourglass. Their task is to shout together when 7 minutes has elapsed. But no one wants to rely too much on the accuracy of their own hourglass. For the first six minutes, everyone is staring at the sand pouring down in their own hourglass. As an agent’s hourglass approaches the end, she lifts her gaze to look around the table to see what the other three are doing. Making eye-contact gives her confidence that the end is near. But if no one else is looking up, she doubts her own timer and delays shouting. On the other hand, if an agent hears her peers shouting, she will ignore her own timer and shout along with them. The authors call this process “reaching consensus“.
But how does the cell-intrinsic timer work? And how can an internal molecular process lead to a burst of electric activity in the absence of any excitatory synaptic inputs? For anyone that knows how photo-transduction works, it should not be hard to imagine ways that a neuron’s membrane potential can be controlled by molecular events. But the beauty of this study is that it really gets to the bottom of things. They were able to trace an outline of the molecular events that implement the timer.
The timer actually consists of two shorter timers, where the conclusion of the first sets off the second. The first timer is a fully cell-intrinsic 5-6 minute timer implemented by CAMKII molecules. That is the subject of their first paper (Thornquist et al 2020). CaMKII is an ancient molecular structure that can store a memory in its phosphorylation state. CAMKII molecules are phosphorylated at the onset of copulation. With the passage of time, they gradually get dephosphorylated and that allows the second timer to begin running.
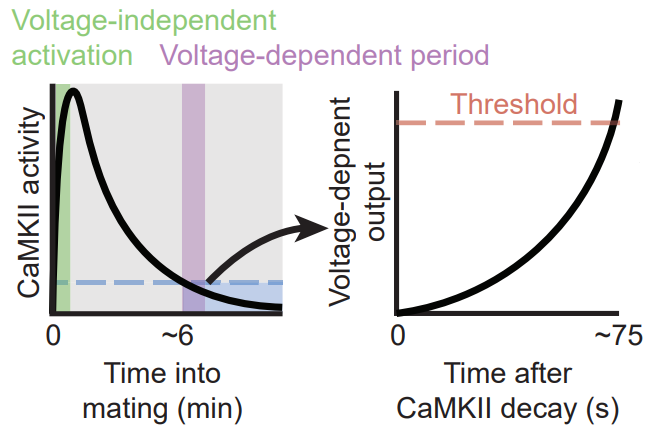
The second timer implements “reaching-consensus”. Although it doesn’t strictly need external inputs to work, it is sped up by inputs from other Corazonin neurons. The main component of this timer is the gradual conversion of ATP to cyclic AMP (cAMP). Through a molecular cascade that follows cAMP production, the cell’s membrane potential rises and this lead to spiking. Normally it takes 60-75 seconds for this timer to lead to the eruption event. But this process can be artificially induced by using a light-gated protein that hastens the production of cAMP in the cell.
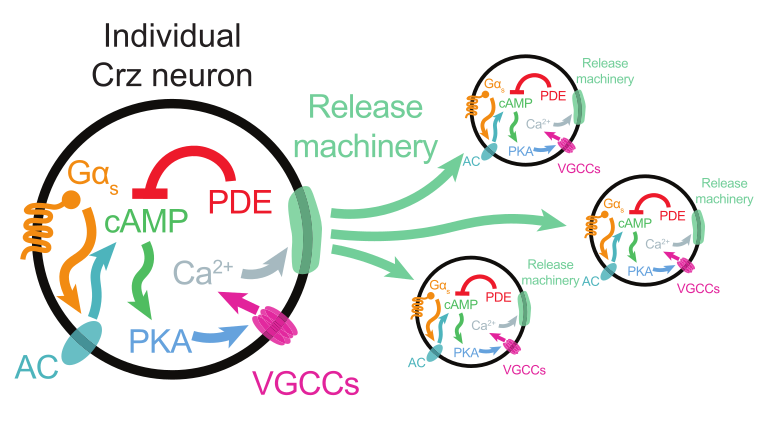
There are still a few missing pieces to the puzzle. For example it is not entirely clear how the CaMKII timer is initiated or how the first timer sets off the second timer. But the general picture has already emerged with serious implications for the entire field. Most of our experimental methods of studying neuronal states (from calcium imaging to fMRI) involve direct or indirect measurements of electric signaling. But here, we have a short-term memory of elapsed time that is undetectable by these methods, because it is stored in the cell’s CaMKII phosphorylation rate and cAMP levels.
Is this a weird hack in flies? Or is it a general time-keeping mechanism for all animals? As someone who has switched from mammalian neuroscience to insect neuroscience, I know there is a tendency in the field to disregard biological discoveries in fruit flies as specializations that cannot be generalized. After all, the fly has fewer neurons at its disposal and cannot afford large networks that produce oscillatory or sequential activity for time-keeping. Why would larger animals need to implement timers with molecules when they have so many neurons?
But I like to turn that question around. If it is possible to implement molecular timers at the scale of minutes or seconds, why would larger organisms ever evolve neural networks for the job? There are a number of serious challenges in implementing timers with electric signalling and computing with molecules is much more efficient in terms of energy, stability, and space. As Sterling & Laughlin put it, “wherever possible, neurons follow the principle: compute with chemistry“. I think it will only be a matter of time before people discover that molecular timers are the norm, rather than the exception.
Dislaimer: Stephen Thornquist is a friend a colleague currently working in the same lab as me. Although to be fair I was tweeting excitedly about his paper before I knew him personally.